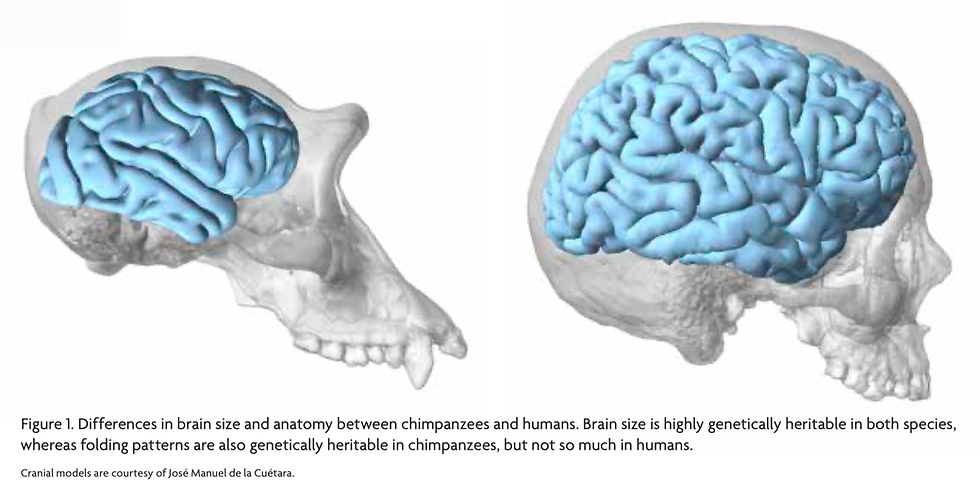
Introduction
The expansion in brain size has always been associated with our increased cognitive abilities and psychological functions. And as we commonly view the intelligence of our species to be the central reason for our success, the process of brain evolution in the hominin lineage has become one of the most crucial topics in the discussion of what constitutes the uniqueness of H. sapiens. However, because of the nature of paleoanthropology, our understanding of the brains of early hominins has been largely limited to the fossil crania discovered and their endocranial cavities. Most of the discussions have been therefore focusing on the gradual increase in endocranial volumes (ECV) or brain size along the hominin lineage.
Nevertheless, brain size is not the only information we can get from these fossils. As the skulls accommodate the brain in the developmental process, there will be imprints of brain convolutions and meningeal vessels on the inner surface (Richtsmeier et al. 2006), which have offered us a precious opportunity to study the evolutionary changes in the structure and developmental pattern of the brain. I consider this incorporation of the evolutionary-developmental (evo-devo) perspective into the study of hominin brain evolution is extremely valuable. As I will argue in the following discussion, the process of hominin brain evolution is remarkably complicated, and ECV by itself might not be a good enough measure for us to understand some of the complex phenomena observed. Instead, changes in brain organization, neural developmental pattern, and neural plasticity might have played a more crucial role in leading to the formation of the modern human brains than we used to think.
Beyond Brain Size
The first critical step is to recognize that the increase in brain size was not a universal trend throughout the hominin evolution (Montgomery 2018). In fact, both the more recently discovered H. naledi in South Africa and the small-bodied hominin H. floresiensis on an island of Indonesia lie outside of this simplistic model.
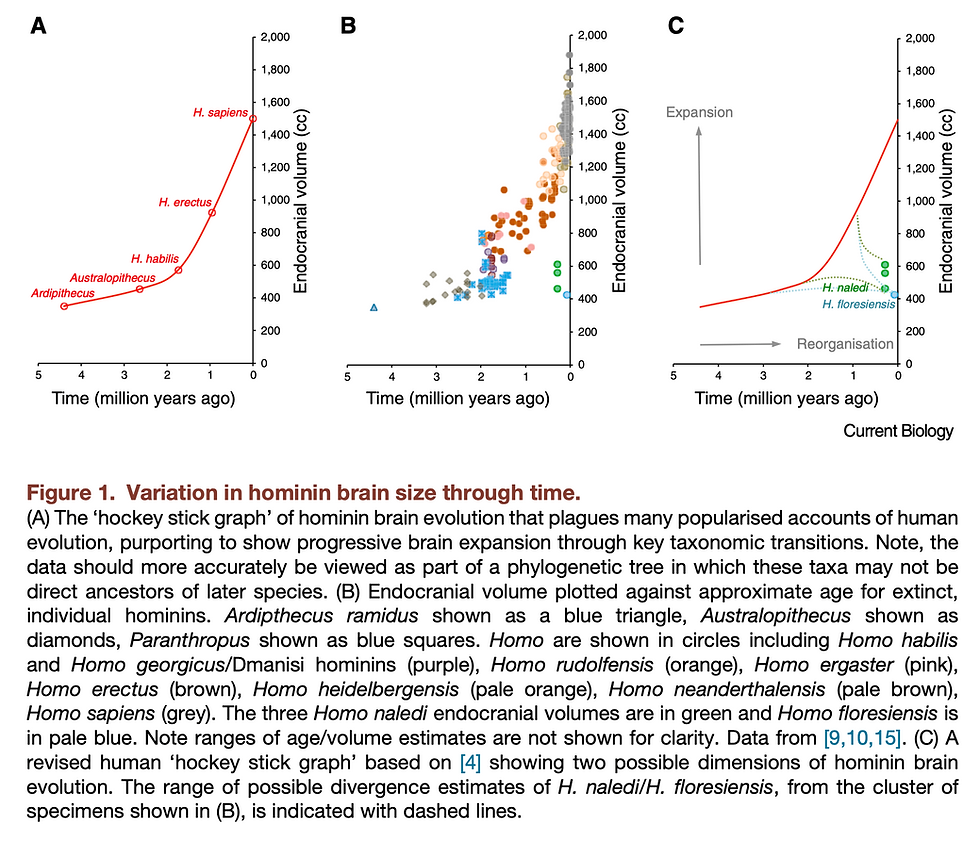
Taking H. naledi as an example, it exhibits a mosaic combination of more derived traits and more primitive traits similar to Australopithecus. While being dated to be around 236 – 335 ka (Dirks et al. 2017), it has a brain size of only around 465 – 610 cc, within the range of Australopithecus (Berger et al. 2015). Whether this small brain size was derived from a reduction event from some Homo species that had larger brains or was it a feature retained from the last common ancestor of the genus Homo remains a difficult question to be answered. However, interestingly, there are some features discovered from the endocasts of H. naledi that seem to be shared with later, larger-brained Homo species. For instance, no fronto-orbital sulcus was observed, which is one of the most notable differences between early hominins (e.g. Australopithecus) and later ones (e.g. H. rudolfensis, H. habilis) in the frontal lobe (Holloway et al. 2018). Combining with its prominent pars orbitalis and high occipital petalial asymmetry, potential enlargement in the frontal lobes and reorganization in the inferior frontal and lateral orbital gyri could be inferred, which might be critical to changes in cognitive and social information processing abilities.
Although the exact phylogenetic and brain morphological details are not yet definitive, similar cases have implied the possibility of decoupling between changes in brain size and structure reorganization, against a simplistic trend of brain expansion. Also supporting this argument, some have argued for brain reorganization in australopithecines prior to the increase in brain size (Falk 2014). And there was evidence showing that the globular shape of the modern human brain might still be evolving gradually until 100,000-35,000 years ago, after the time when brain size was already in the range of modern humans around 300,000 years ago (Neubauer, Hublin, and Gunz 2018). This globularization process will be further discussed in later parts of this essay, but for now, it also serves as a piece of strong evidence for how natural selection could act upon the shape and structure of the cortices independently, without necessarily altering the overall brain size.
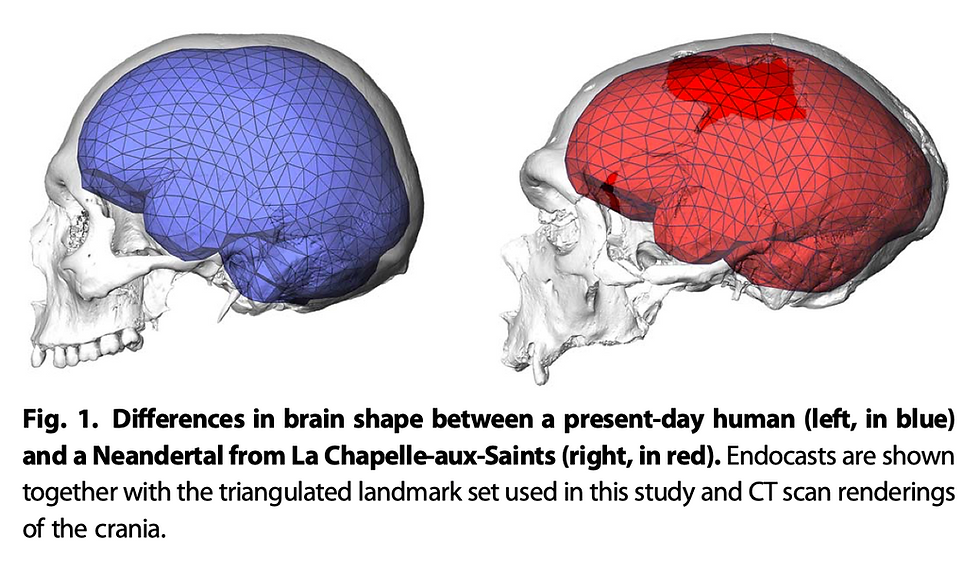
Neural Ontogeny and Plasticity
While we usually use the mature brains of adults in our comparisons and analyses, the brain ontogenetic pattern and the associated changes in life history are also important for us to interpret the mechanisms underlying the formation of these “final products” we see.
Neurodevelopment is a remarkably complex process (Tau and Peterson 2010). From early gastrulation and neuronal proliferation to later neural migration, from extensive synaptogenesis to pruning, axon elimination, and apoptosis, every step of brain development is highly organized and requires both genetic predispositions and environmental inputs to function properly. Therefore, unsurprisingly, when we compare the neural ontogeny of H. sapiens with those of chimpanzees and other hominins, it is clear that we exhibit multiple unique features in terms of the spatiotemporal pattern.
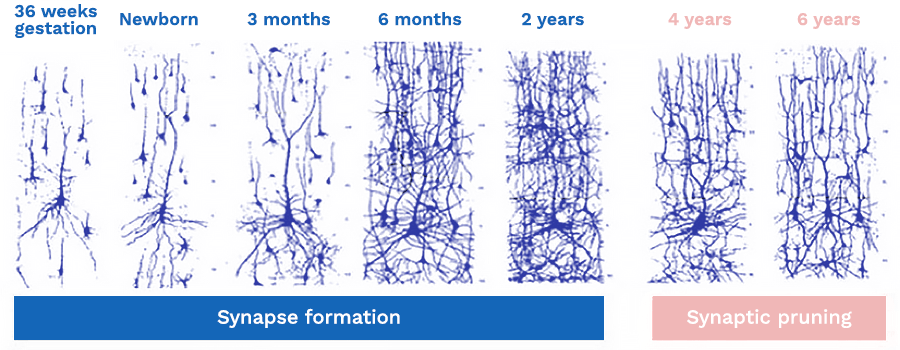
H. sapiens has a prolonged process of brain development. While other primates are relatively precocial, human newborns are extremely altricial, and the neonatal brain size is only around a quarter of adult brain size, comparing to around 40% for chimpanzees and orangutans (Gómez-Robles and Sherwood 2016). This is probably a result of both obstetrical (Rosenberg 1992) and metabolic constraints (Dunsworth et al. 2012) during the process of brain expansion. However, this also means that human infants will experience high rates of postnatal brain growth and are inevitably exposed to environmental and cultural inputs for a longer period, which may exert a large influence on the formation of neural circuitry and connectivity (Gómez-Robles and Sherwood 2016).
Comparing with chimpanzees, we are shown to have protracted neocortical myelination that extends beyond late adolescence, which facilitates saltatory conduction and is central to the communication between neurons (Miller et al. 2012; Sakai et al. 2013). Furthermore, the cortical organization was found to be less genetically heritable in humans than in chimpanzees, which may suggest higher plasticity and dynamics involved in the growth of the human brain (Gómez-Robles et al. 2015).
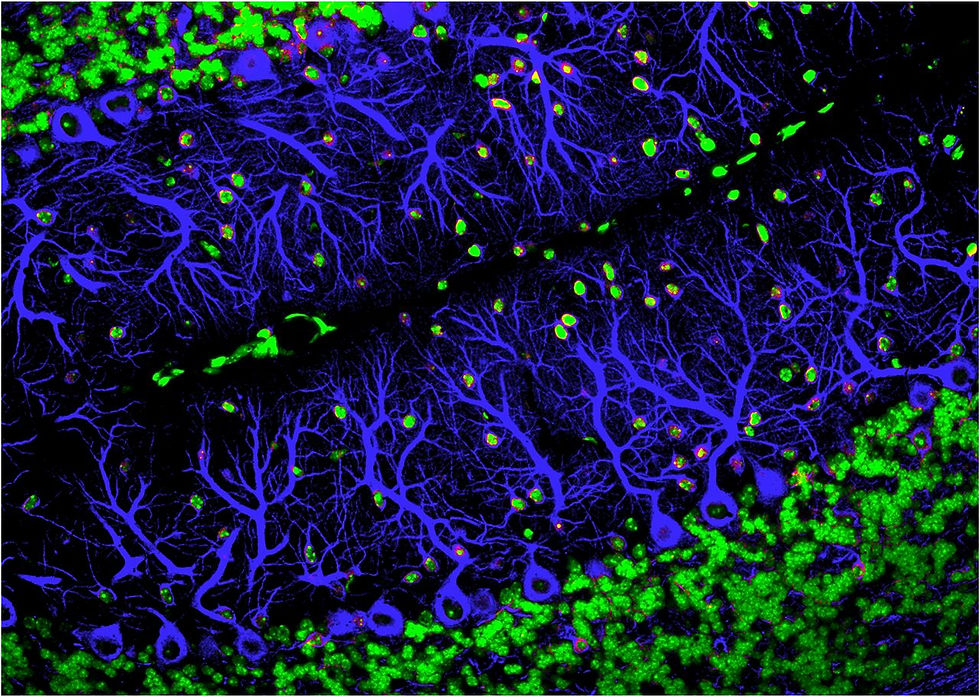
Although it is challenging to reconstruct the process of neonatal development in extinct hominins comparing to conducting comparative studies with chimpanzees, some researchers have tried to analyze the neural development in H. erectus and Neanderthals. For instance, some have focused on the infant specimen of H. erectus from Mojokerto and found its absolute brain growth rates to be higher than chimpanzees and at the lower end of modern humans (Cofran and DeSilva 2015). According to the analysis, the authors have also suggested secondary altriciality in H. erectus, which has great implications for key human adaptations of parental care and cooperative breeding. For Neanderthals, Gunz et al. (2010) have done a series of studies demonstrating the uniqueness of an early postnatal globularization stage observed in H. sapiens but absent in Neanderthals. Although the meaning and mechanisms behind this globularization stage are still inconclusive, it is highly likely that it involves further modifications on the cortical organization and serves as a part of the explanation for the evolution of human intelligence and culture.
To summarize, the evo-devo approach has showcased the significance of changes in cortical organization and plasticity throughout the hominin evolution process. Brain evolution was not simply an allometric expansion of brain size. Instead, to understand evolution on the level of cognitive functions, we must base our investigations on the most appropriate level – the organization of cortical structures.
Insights from Ancient DNA
Ultimately, evolution and natural selection act on genes, and almost all phenotypic changes involve modifications on the genetic level. Brain evolution is certainly one of them. Following the rapid development of molecular biology and genome sequencing technology in the last few decades, numerous comparisons between human and other primate genomes have been carried out. Although ancient DNA data have been largely limited to the ones for Neanderthals and Denisovans, scientists have still found multiple genes that might have contributed to this extremely complicated process.
For example, some genes that are related to microcephaly – a disorder characterized by infants having significantly reduced brain sizes – have been indicated to be crucial contributors to the evolutionary expansion of the brain. These genes include ASPM, MCPH1, etc., and research has provided evidence for them to be the target of strong positive selection during primate evolution. As both ASPM and MCPH1 have regulatory functions on the process of neuronal proliferation, changes in terms of mutation or expression pattern of these genes could all potentially result in an increase in brain size and neuron number (Gilbert, Dobyns, and Lahn 2005).

Another important gene is FOXP2, which is a transcription factor discovered to be involved in speech and language production (Lai et al. 2001). Interestingly, while the human version of FOXP2 has two amino acid differences from the version for chimpanzees, gorillas, and rhesus macaques (Enard et al. 2002), the same version has been found in Neanderthals and Denisovans (Krause et al. 2007; Reich et al. 2010). This might have some implications for the FOXP2-related plasticity and language capacity among Neanderthals, Denisovans, us, and our last common ancestor. However, as language is a complex function that likely involves multiple coordinated changes in brain connectivity and other modalities, these implications remain suggestive only.
Conclusion
In this paper, I have argued that hominin brain evolution is a lot more than the expansion of brain size. Instead, the importance of changes in brain organization, neural developmental pattern, and neural plasticity cannot be ignored. Despite the practical difficulties involved in studying the cortical structures and developmental patterns of ancient hominins, I believe further research with the evo-devo approach and the invention of new technologies will provide us with more converging evidence from different fields and help delineate a more coherent theory for hominin brain evolution.
References
Berger, Lee R, John Hawks, Darryl J de Ruiter, Steven E Churchill, Peter Schmid, Lucas K Delezene, Tracy L Kivell, et al. 2015. “Homo Naledi, a New Species of the Genus Homo from the Dinaledi Chamber, South Africa.” ELife 4 (September): e09560. https://doi.org/10.7554/eLife.09560.
Cofran, Zachary, and Jeremy M. DeSilva. 2015. “A Neonatal Perspective on Homo Erectus Brain Growth.” Journal of Human Evolution 81 (April): 41–47. https://doi.org/10.1016/j.jhevol.2015.02.011.
Dirks, Paul HGM, Eric M Roberts, Hannah Hilbert-Wolf, Jan D Kramers, John Hawks, Anthony Dosseto, Mathieu Duval, et al. 2017. “The Age of Homo Naledi and Associated Sediments in the Rising Star Cave, South Africa.” ELife 6 (May): e24231. https://doi.org/10.7554/eLife.24231.
Dunsworth, Holly M., Anna G. Warrener, Terrence Deacon, Peter T. Ellison, and Herman Pontzer. 2012. “Metabolic Hypothesis for Human Altriciality.” Proceedings of the National Academy of Sciences 109 (38): 15212–16. https://doi.org/10.1073/pnas.1205282109.
Enard, Wolfgang, Molly Przeworski, Simon E. Fisher, Cecilia S. L. Lai, Victor Wiebe, Takashi Kitano, Anthony P. Monaco, and Svante Pääbo. 2002. “Molecular Evolution of FOXP2 , a Gene Involved in Speech and Language.” Nature 418 (6900): 869–72. https://doi.org/10.1038/nature01025.
Falk, Dean. 2014. “Interpreting Sulci on Hominin Endocasts: Old Hypotheses and New Findings.” Frontiers in Human Neuroscience 8. https://doi.org/10.3389/fnhum.2014.00134.
Gilbert, Sandra L., William B. Dobyns, and Bruce T. Lahn. 2005. “Genetic Links between Brain Development and Brain Evolution.” Nature Reviews Genetics 6 (7): 581–90. https://doi.org/10.1038/nrg1634.
Gómez-Robles, Aida, William D. Hopkins, Steven J. Schapiro, and Chet C. Sherwood. 2015. “Relaxed Genetic Control of Cortical Organization in Human Brains Compared with Chimpanzees.” Proceedings of the National Academy of Sciences 112 (48): 14799–804. https://doi.org/10.1073/pnas.1512646112.
Gómez-Robles, Aida, and Chet C. Sherwood. 2016. “Human Brain Evolution: How the Increase of Brain Plasticity Made Us a Cultural Species.” Mètode Revista de Difusió de La Investigació 0 (7). https://doi.org/10.7203/metode.7.7602.
Gunz, Philipp, Simon Neubauer, Bruno Maureille, and Jean-Jacques Hublin. 2010. “Brain Development after Birth Differs between Neanderthals and Modern Humans.” Current Biology 20 (21): R921–22. https://doi.org/10.1016/j.cub.2010.10.018.
Holloway, Ralph L., Shawn D. Hurst, Heather M. Garvin, P. Thomas Schoenemann, William B. Vanti, Lee R. Berger, and John Hawks. 2018. “Endocast Morphology of Homo Naledi from the Dinaledi Chamber, South Africa.” Proceedings of the National Academy of Sciences 115 (22): 5738–43. https://doi.org/10.1073/pnas.1720842115.
Krause, Johannes, Carles Lalueza-Fox, Ludovic Orlando, Wolfgang Enard, Richard E. Green, Hernán A. Burbano, Jean-Jacques Hublin, et al. 2007. “The Derived FOXP2 Variant of Modern Humans Was Shared with Neandertals.” Current Biology 17 (21): 1908–12. https://doi.org/10.1016/j.cub.2007.10.008.
Lai, Cecilia S. L., Simon E. Fisher, Jane A. Hurst, Faraneh Vargha-Khadem, and Anthony P. Monaco. 2001. “A Forkhead-Domain Gene Is Mutated in a Severe Speech and Language Disorder.” Nature 413 (6855): 519–23. https://doi.org/10.1038/35097076.
Miller, Daniel J., Tetyana Duka, Cheryl D. Stimpson, Steven J. Schapiro, Wallace B. Baze, Mark J. McArthur, Archibald J. Fobbs, et al. 2012. “Prolonged Myelination in Human Neocortical Evolution.” Proceedings of the National Academy of Sciences 109 (41): 16480–85. https://doi.org/10.1073/pnas.1117943109.
Montgomery, Stephen. 2018. “Hominin Brain Evolution: The Only Way Is Up?” Current Biology 28 (14): R788–90. https://doi.org/10.1016/j.cub.2018.06.021.
Neubauer, Simon, Jean-Jacques Hublin, and Philipp Gunz. 2018. “The Evolution of Modern Human Brain Shape.” Science Advances 4 (1): eaao5961. https://doi.org/10.1126/sciadv.aao5961.
Reich, David, Richard E. Green, Martin Kircher, Johannes Krause, Nick Patterson, Eric Y. Durand, Bence Viola, et al. 2010. “Genetic History of an Archaic Hominin Group from Denisova Cave in Siberia.” Nature 468 (7327): 1053–60. https://doi.org/10.1038/nature09710.
Richtsmeier, Joan T., Kristina Aldridge, Valerie B. DeLeon, Jayesh Panchal, Alex A. Kane, Jeffrey L. Marsh, Peng Yan, and Theodore M. Cole. 2006. “Phenotypic Integration of Neurocranium and Brain.” Journal of Experimental Zoology Part B: Molecular and Developmental Evolution 306B (4): 360–78. https://doi.org/10.1002/jez.b.21092.
Rosenberg, Karen R. 1992. “The Evolution of Modern Human Childbirth.” American Journal of Physical Anthropology 35 (S15): 89–124. https://doi.org/10.1002/ajpa.1330350605.
Sakai, Tomoko, Mie Matsui, Akichika Mikami, Ludise Malkova, Yuzuru Hamada, Masaki Tomonaga, Juri Suzuki, et al. 2013. “Developmental Patterns of Chimpanzee Cerebral Tissues Provide Important Clues for Understanding the Remarkable Enlargement of the Human Brain.” Proceedings of the Royal Society B: Biological Sciences 280 (1753): 20122398. https://doi.org/10.1098/rspb.2012.2398.
Tau, Gregory Z., and Bradley S. Peterson. 2010. “Normal Development of Brain Circuits.” Neuropsychopharmacology 35 (1): 147–68. https://doi.org/10.1038/npp.2009.115.
Comments